Biomass densification for energy production
Learn about the density properties of solid biomass and potential densification technologies to increase energy efficiency. This technical information is for Ontario producers.
ISSN 1198-712X, Published June 2011
Introduction
Biomass fuels are a potential source of renewable energy. One of the major barriers to their widespread use is that biomass has a lower energy content than traditional fossils fuels, which means that more fuel is required to get the same amount of energy.
When combined — low energy content with low density — the volume of biomass handled increases enormously. Compaction or densification is one way to increase the energy density and overcome handling difficulties.
This factsheet examines the density properties of solid biomass and potential densification technologies.
Bulk density
Bulk density is defined as the weight per unit volume of a material, expressed in kilograms per cubic metre (kg/m3) or pounds per cubic foot (lb/ft3). Most agricultural residues have low bulk densities, as shown in Figure 1.
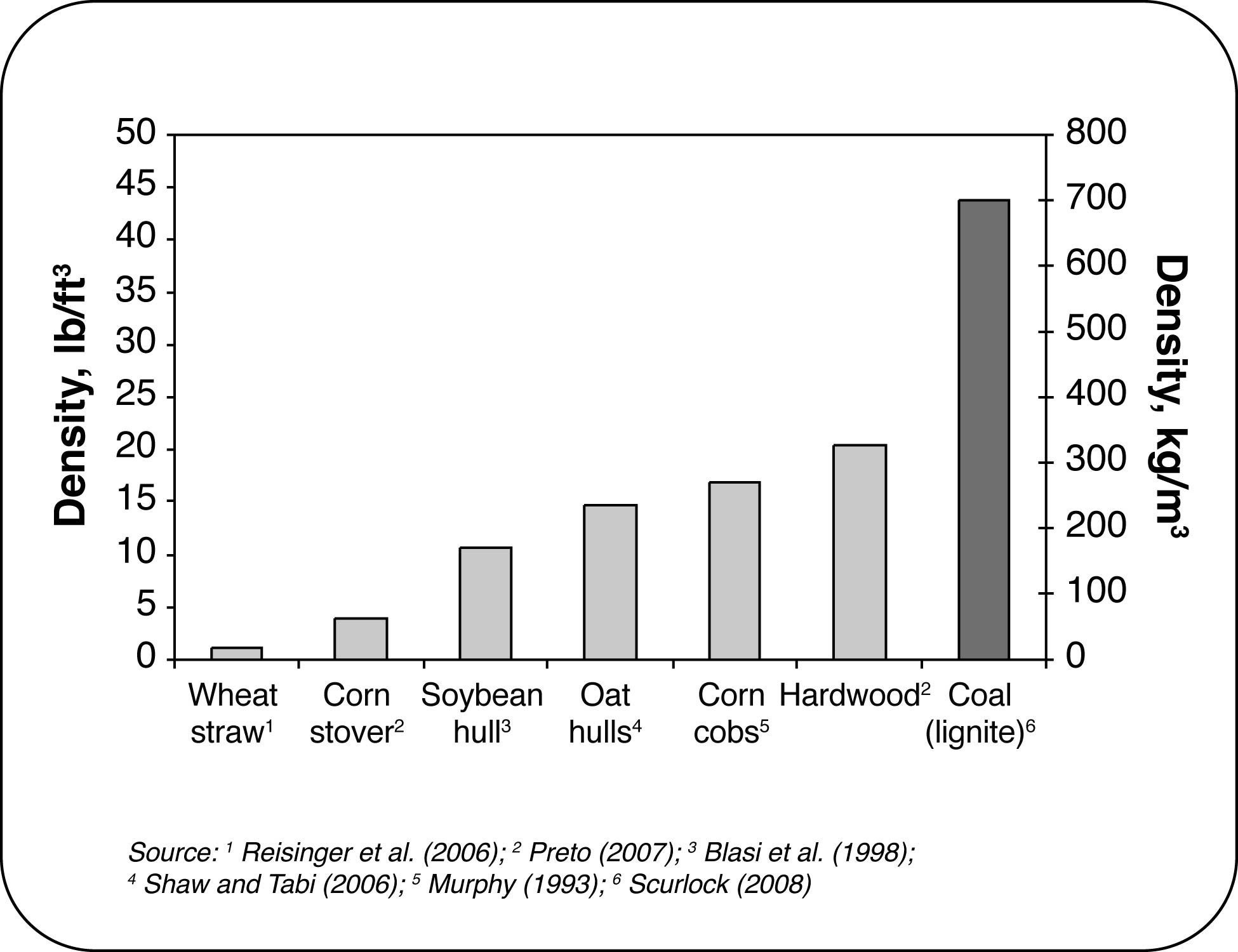
For example, the bulk density of loose wheat straw is approximately 18 kg/m3 (1.1 lb/ft3), in comparison to coal at 700 kg/m3 (44 lb/ft3). For this reason, it generally is only economically feasible to transport unprocessed biomass less than approximately 200 km
Energy density
Energy density is a term used to describe the amount of energy stored per unit volume, often expressed in MJ/m3 or BTU/ft3.
Figure 2 is a graphical representation of common volume ratios for unprocessed material, with the cubes representing the volume of material required for equal energy, 16:4:1 for straw to wood to coal.
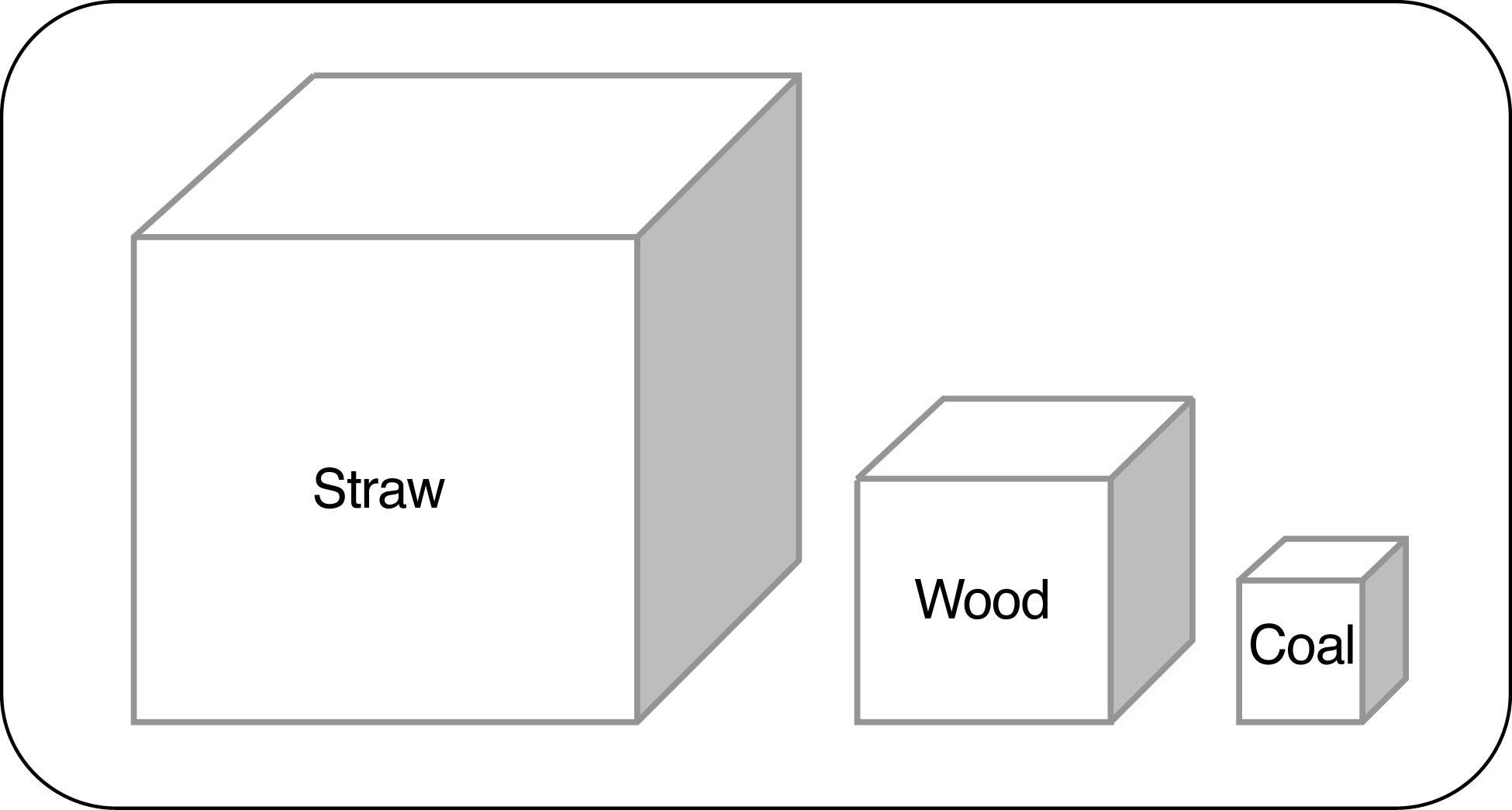
Densify
The low density of biomass materials poses a challenge for the handling, transportation, storage and combustion processes. These problems may be addressed through densification, a process that produces either liquid or solid fuel with denser and more uniform properties than the raw biomass.
The main advantages of biomass densification for combustion are:
- simplified mechanical handling and feeding
- uniform combustion in boilers
- reduced dust production
- reduced possibility of spontaneous combustion in storage
- simplified storage and handling infrastructure, lowering capital requirements at the combustion plant
- reduced cost of transportation due to increased energy density
The major disadvantage to biomass densification technologies is the high cost associated with some of the densification processes.
Pre-treatment of biomass
Prior to biomass densification, pre-treatments may be required to optimize the energy content and bulk density of the product.
Pre-treatment can include:
- chop length/grinding
- drying to required moisture content
- application of a binding agent
- steaming
- torrefaction
Chop length/grinding
Each densification process requires specific chop length and/or grinding to achieve:
- lower energy use in the densification process
- denser products
- a decrease in breakage of the outcome product
footnote iii
Drying
Low moisture results in improved density and durability of the fuel
Addition of a binding agent
The density and durability of densified biomass are influenced by the natural binding agents of the material. The binding capacity increases with a higher protein and starch content
Steaming
The addition of steam prior to densification can aid in the release and activation of natural binders present in the biomass.
Torrefaction
Torrefaction is a version of pyrolysis processes that comprise the heating of biomass in the absence of oxygen and air. Torrefaction is a pre-treatment process used to improve the properties of pellets. It can also be used as a stand-alone technique to improve the properties of biomass. Torrefaction is a mild version of slow pyrolysis in which the goal is to dry, embrittle and waterproof the biomass. This is accomplished by heating the biomass in an inert environment at temperatures of 280°C–320°C.
Techniques for biomass densification
Biomass is densified via two main processes: mechanical densification and pyrolysis. Mechanical densification involves applying pressure to mechanically densify the material. Pyrolysis involves heating the biomass in the absence of oxygen. In general, lower temperatures at longer processing times (i.e., slow pyrolysis) favour solid (charcoal) production. Medium temperatures (400°C–500°C) at very short times (1–2 seconds), known as fast pyrolysis, favour liquid or bio-oil production.
The method of densification depends on the type of residues and the local situation. Table 1 outlines the various technologies used to increase the biomass energy density and/or mould the fuel into a homogeneous size and shape.
![]() |
Mechanical densification – Bales are a traditional method of densification commonly used to harvest crops. A bale is formed using farm machinery (called a baler) that compresses the chop. Bales can be square, rectangular or round, depending on the type of baler used. The dimensions of round bales range from 1.2 m x 1.5 m (4 ft x 5 ft) to 1.5 m x 1.5 m (5 ft x 5 ft). Large rectangular bales typically measure 0.9 m x 0.9 m x 1.8 m (3 ft x 3 ft x 6 ft) in length. Round bales are less expensive to produce, however, large square bales are usually denser and easier to handle and transport. |
![]() |
Mechanical densification - Pellets are very high in density. They are easier to handle than other densified biomass products, since infrastructure for grain handling is used for pellets. Pellets are formed by an extrusion process, using a piston press, where finely ground biomass material is forced through round or square cross-sectional dies and cut to a desired length. The standard shape of a biomass pellet is a cylinder, having a length smaller than 38 mm (1.5 in.) and a diameter around 7 mm (0.3 in.). Although uniform in shape, pellets are easily broken during handling. Different grades of pellets vary in energy and ash content. Photo courtesy of CanmetENERGY. |
![]() |
Mechanical densification - Cubes are larger pellets, usually square in shape. Cubes are less dense than pellets. Cube sizes range from 13–38 mm (0.5–1.5 in.) in cross section, with a length ranging 25–102 mm (1–4 in.). The process involves compressing chopped biomass with a heavy press wheel, followed by forcing the biomass through dies to produce cubes. Photo courtesy of CanmetENERGY. |
![]() |
Mechanical densification - Briquettes are similar to pellets but differ in size. Briquettes have a diameter of 25 mm (1 in.) or greater and are formed when biomass is punched, using a piston press, into a die under high pressure. Alternatively, a process referred to as screw extrusion can be used. In screw extrusion, the biomass is extruded by a screw through a heated die. Biomass densified through screw extrusion has higher storability and energy density properties compared to biomass produced by piston press. Photo courtesy of Wayne Winkler. |
![]() |
Mechanical densification - Pucks are similar in appearance to a hockey puck, with a 75 mm (3 in.) diameter. They are produced using a briquetter and are resilient to moisture. Pucks have a similar density as pellets, with the advantage that they require lower production costs compared to pelletization. |
![]() |
Mechanical densification - Wood chips are used in many operations, from household appliances to large-scale power plants. Woodchips for boilers range in size, 5–50 mm (0.2–2 in.) in length. Woodchips are made with a woodchipper. In terms of fuel, woodchips are comparable in cost to coal. Photo courtesy of CanmetENERGY. |
![]() |
Pyrolysis - Torrefaction is carried out by heating biomass in an inert atmosphere at temperatures of 280°C–320°C for a few minutes. The torrefied fuel shows improved grindability properties. Torrefied biomass has hydrophobic properties (repels water), making it resistant to biological attack and moisture, thereby facilitating its storage. The process requires little energy input since some of the volatile gases liberated during heating are combusted, generating 80% of the heat required for torrefaction. Torrefied biomass is densified into pellets or briquettes, further increasing the density of the material and improving its hydrophobic properties. Photo courtesy of CanmetENERGY. |
![]() |
Pyrolysis - Slow pyrolysis involves heating biomass to 350°C–500°C in the absence of oxygen and air for extended periods of time (typically 0.5–2 hours). The principal product is a solid (charcoal) that retains 60%–70% of the original energy from the raw biomass. The energy density can be increased, and thus charcoal is a suitable fuel for commercial uses similar to torrified biomass, residential use, i.e., barbecues, and as a potential soil improvement additive known as bio-char. Photo courtesy of CanmetENERGY. |
![]() |
Pyrolysis - Fast pyrolysis involves processing biomass at temperatures of up to 450°C–500°C for 1–2 seconds. The process yields up to 75% bio-oil and 10%–15% charcoal. Bio-oil is a higher-energy density fuel, and its handling properties are simplified, as the fuel is a liquid that is pumped and stored in tanks. Precautions are necessary, as bio-oils are very acidic, have a pungent odour and are prone to separation/settling. Substitute bio-oil for fossil fuel, heavy and middle oils. Research is under way to explore conversion to lighter oils such as diesel and gasoline. Photo courtesy of CanmetENERGY. |
Through various densification technologies, raw biomass is compressed to densities in the order of 7–10 times its original bulk density
Form of biomass | Shape and size characteristics | Density (lb/ft3) |
Density (kg/m3) |
Energy density (GJ/m3) |
---|---|---|---|---|
Traditional method Baled biomass |
Large round, Soft core 1.2 x 1.2, 1.2 x 1.5, 1.5 x 1.2, 1.8 x 1.5 m (4 x 4, 4 x 5, 5 x 4, 6 x 5 ft) diameter x width |
10-12 | 160-190 | 2.8-3.4 |
Traditional method Baled biomass |
Large round, Hard core 1.2 x 1.2, 1.2 x 1.5, 1.5 x 1.2, 1.8 x 1.5 m (4 x 4, 4 x 5, 5 x 4, 6 x 5 ft) diameter x width |
12–15 | 190–240 | 3.4–4.5 |
Traditional method Baled biomass |
Large/Mid-size square 0.6 x 0.9 x 2.4 m (2 x 3 x 8 ft) 0.9 x 1.2 x 2.4 m (3 x 4 x 8 ft) |
13–16 | 210–255 | 3.7–4.7 |
Non-traditional method Ground biomass (i.e., hammermill) |
1.5 mm (0.06 in.) pack fill with tapping | 13 | 200 | 3.6 |
Non-traditional method Briquettes |
32 mm (1.3 in.) diameter x 25 mm (1 in.) thick | 22 | 350 | 6.4 |
Non-traditional method Cubes |
33 mm (1.3 in.) x 33 mm (1.3 in.) cross section | 25 | 400 | 7.3 |
Non-traditional method Pucks |
75 mm (3 in.) diameter x 12 mm (0.5 in.) thick | 30–40 | 480–640 | 8.6–12.0 |
Non-traditional method Pellets |
6.24 mm (0.2 in.) diameter | 35–45 | 550–700 | 9.8–14.0 |
Non-traditional method Torrefied pellets |
6.24 mm (0.2 in.) diameter | 50 | 800 | 15.0 |
Non-traditional method Bio-oil |
liquid | 75 | 1,200 | 20 |
Conversion from | Conversion to | Multiply by |
---|---|---|
mm | inch | 0.0394 |
inch | ft | 0.0833 |
kg/m3 | lb/ft3 | 0.0624 |
MJ/kg | BTU/lb | 430 |
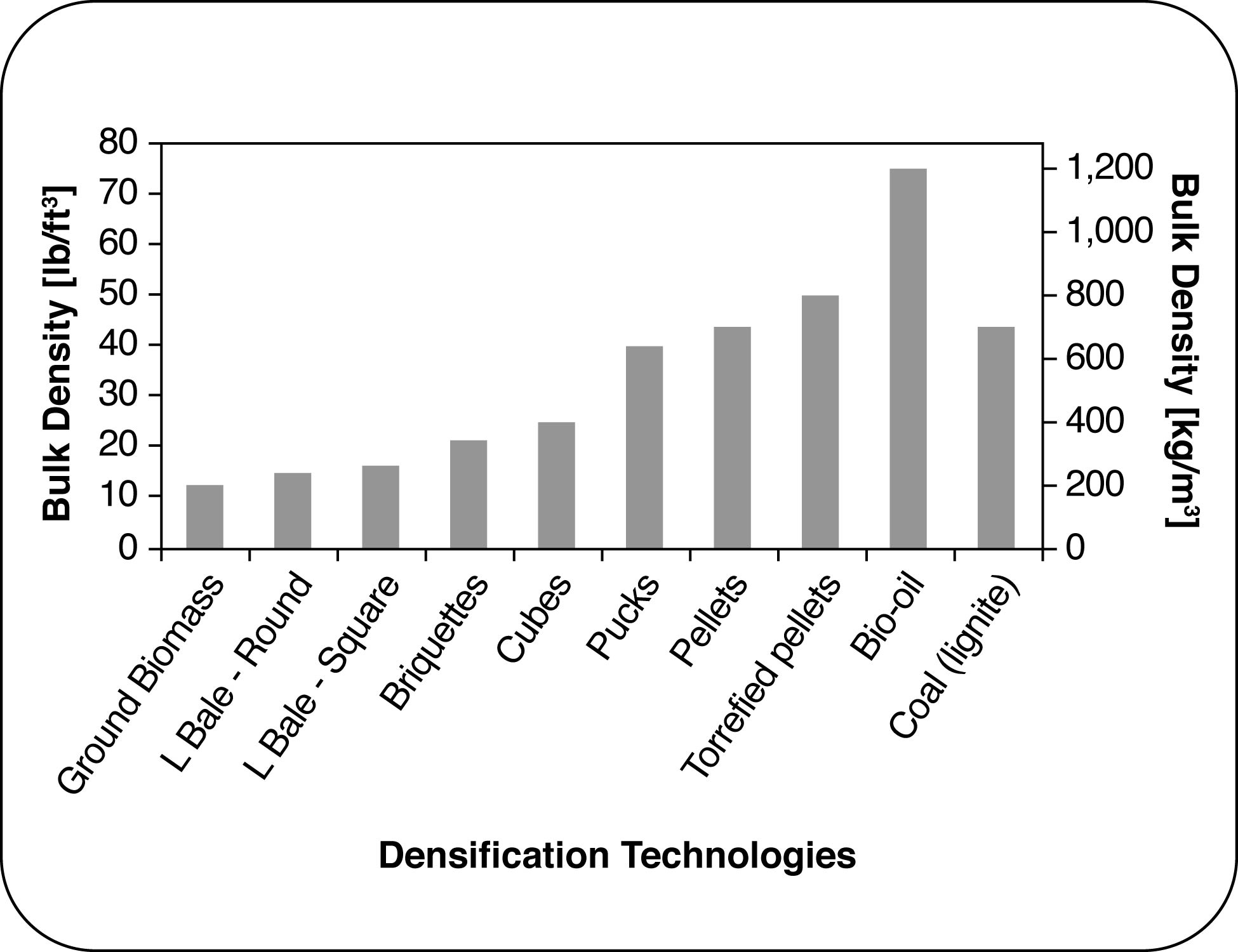
Biomass densification cost
Pyrolyzed materials are the most expensive to densify, with cubes, pucks, briquettes and woodchips being less expensive.
Factors affecting the cost of densification technologies include
- size of densification plant (tonnes/year)
- operating time (hours/day)
- equipment cost
- personnel cost
- raw material costs
Densification technologies result in higher energy inputs and increased costs. A portion of the cost is recuperated by the lower handling, storage and transportation costs, and better operability of the boiler and combustion process. Some densification technologies mentioned are commercially available, while others are emerging.
Conclusion
The low-energy density of biomass by volume, in comparison with fossil fuels, results in higher handling, storage and transportation costs. Consequently, biomass is most economically feasible when used close to the source. The cost of biomass transportation is reduced through densification technologies. Densification technologies produce a homogeneous product with a higher energy density than that of the original raw material, at the expense of new capital and operating costs.
Resources
This factsheet was written by Steve Clarke, P.Eng. (retired), engineer, energy and crop engineering specialist, OMAFRA, Kemptville, and Fernando Preto, bioenergy systems, CanmetENERGY. A special thanks is extended to Chantal Quesnel for all of her hard work in helping create this fact sheet, Benjamin Bronson for his contributions and conscientious review and Shalin Khosla, greenhouse specialist, OMAFRA, for his considerable assistance in evaluating alternate technologies.
Footnotes
- footnote[i] Back to paragraph Preto, F. (2007). Strategies and techniques for combustion of agricultural biomass fuels. Growing the Margins Energy Conference.
- footnote[ii] Back to paragraph Preto, F. (2007). Strategies and techniques for combustion of agricultural biomass fuels. Growing the Margins Energy Conference.
- footnote[iii] Back to paragraph Dobie, J.B. (1959). Engineering appraisal of hay pelletingn . Agricultural Engineering, 40(2), 72–76.
- footnote[iv] Back to paragraph Shaw, M., and Tabil, L. (2007). Compression and relaxation characteristics of selected biomass grinds. ASABE Paper No. 076183. St. Joseph, Mich.: ASABE
- footnote[v] Back to paragraph Kaliyan, N., Morey, R.V. (2009).Factors affecting strength and durability of densified biomass products. Biomass Bioenergy 33 (3), 337–359.
- footnote[vi] Back to paragraph Tabil, L.G., Sokhansani, S., and Tyler, R.T. (1997). Performance of different binders during alfalfa pelleting. Canadian Agricultural Engineering, 39(1), 17–23.
- footnote[vii] Back to paragraph Kaliyan, N., Morey, V. (2006). Densification characteristics of corn stover and switchgrass. ASABE Paper No. 066174. St. Joseph, Mich.: ASABE.
- footnote[viii] Back to paragraph Demirbas, K., Sahin-Demirba, K.A. (2009). Compacting of biomass for energy densification. Energy Sources, Part A: Recovery, Utilization and Environmental Effects. 1556–7230, 31(12), 1063–1068.
- footnote[ix] Back to paragraph Mani, S., Sokhansanj, S., Bi, X., and Turhollow, A. (2006). Economics of producing fuel pellets from biomass. Applied Engineering in Agriculture, 22(3), 421–26.
- footnote[x] Back to paragraph Mani, S., Sokhansanj, S., Bi, X., and Turhollow, A. (2006). Economics of producing fuel pellets from biomass. Applied Engineering in Agriculture, 22(3), 421–26.
- footnote[xi] Back to paragraph Mani, S., Sokhansanj, S., Bi, X., and Turhollow, A. (2006). Economics of producing fuel pellets from biomass. Applied Engineering in Agriculture, 22(3), 421–26.
- footnote[xii] Back to paragraph Winkler, W. (2010). Briquetting Systems. New York.
- footnote[xiii] Back to paragraph Mani, S., Sokhansanj, S., Bi, X., and Turhollow, A. (2006). Economics of producing fuel pellets from biomass. Applied Engineering in Agriculture, 22(3), 421–26.
- footnote[xiv] Back to paragraph Kiel, J. (2007). Torrefaction for biomass upgrading into commodity fuels. Presentation to IEA Bioenergy Task 32 Workshop "Fuel storage, handling and preparation and system analysis for biomass combustion technologies." Berlin Germany, 7 May 2007.
- footnote[xv] Back to paragraph Kiel, J. (2007). Torrefaction for biomass upgrading into commodity fuels. Presentation to IEA Bioenergy Task 32 Workshop "Fuel storage, handling and preparation and system analysis for biomass combustion technologies." Berlin Germany, 7 May 2007.
- footnote[xvi] Back to paragraph Mani, S., Sokhansanj, S., Bi, X., and Turhollow, A. (2006). Economics of producing fuel pellets from biomass. Applied Engineering in Agriculture, 22(3), 421–26